The Evolution of Loudspeaker Measurement
(or the futility of using 20th century tools to address 21st century needs)
By Tim Gladwin
Today’s hyper-competitive loudspeaker market puts product development teams under intense pressure to innovate and improve product performance while lowering development time and costs. And yet product developers are often saddled with mid-20th century tools that are expensive, slow and inaccurate.
In this paper we will briefly review the history of loudspeaker measuring tools, their advantages and disadvantages, and compare them to a new measurement device from noted acoustic measurement pioneer Klippel GmbH. Klippel’s new Near Field Scanner (NFS) promises lower costs, fewer man-hours and greater accuracy than any other method.
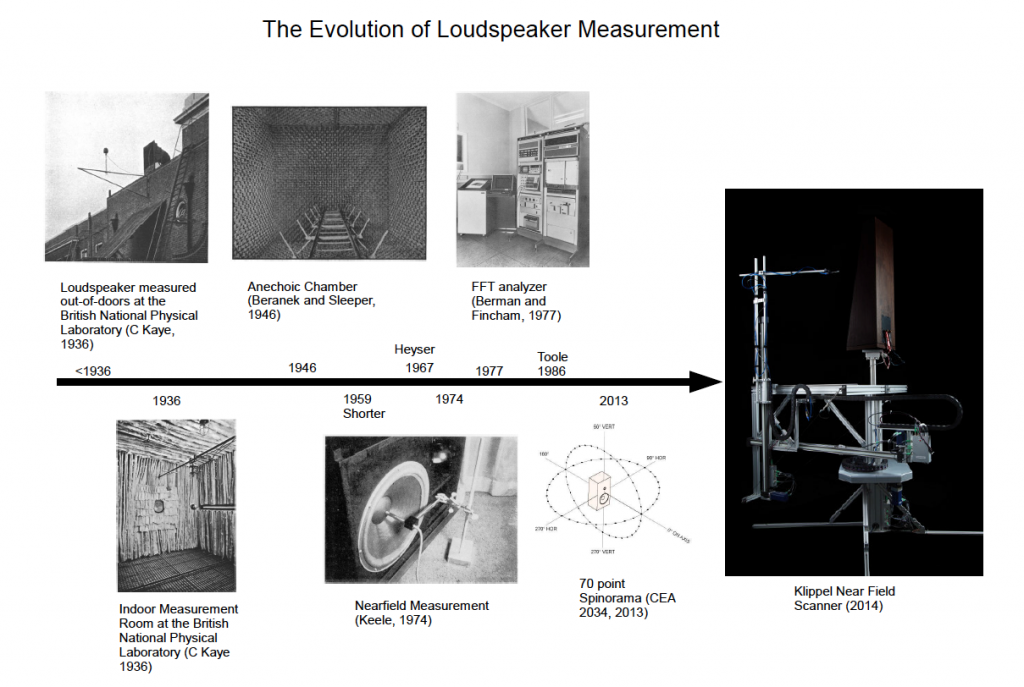
Anechoic chambers have been used to provide a controlled environment for loudspeaker measurement since the early 1930’s. They were developed to reduce the limitations of outdoor measurements: extraneous noise and weather. By 1946, Beranek and Sleeper had optimized wedge and chamber design (JASA 18-1 1946). Today’s anechoic chambers are essentially unchanged from Baranek & Sleeper’s time.
At the time, the test signal was a slow swept sine wave from a heterodyne oscillator, often recorded directly to paper on a strip chart recorder. The ballistics of the pen and the speed of the sweep provided smoothing.
The on-axis SPL curve has traditionally been one of the key measures of the transfer function of the loudspeaker, but by the 50’s it was clear that the entire acoustic output must be measured to determine the true transfer function (Shorter, JAES 7-1, 1959). Turntables in anechoic chambers generated polar measurements. This is a slow process and there is the difficulty cantilevering a speaker about its reference axis with great precision, making this method prone to error.
The high cost of building chambers large enough to make meaningful free-field measurements of entire systems, particularly at frequencies below 100Hz*, remains a significant disadvantage. Most engineers apply a correction curve to anechoic chamber measurements. Those curves are often based on a measurement of a single speaker and for a single position of the microphone and source, introducing further opportunity for error. The irregular absorption/reflection character (anisotropy) of anechoic chambers is a real and key factor that inhibits reliable polar measurements.
Richard Heyser introduced new measurement techniques of time delay spectroscopy and Fourier analysis in 1967 and 1969. This laid the groundwork for advances in non-anechoic measurement. In 1974 Don Keele demonstrated that key loudspeaker measurements could be made in the near field. The advent of digital computers led Berman and Fincham to adapt FFT analyzers for acoustic measurement in 1973. These advances allowed for new stimuli; a slow swept sine signal was no longer required. The impulse response had been used in laboratories since the 40’s, but more powerful computers made the impulse response a viable alternative to the slow sine sweep. Gating of the signal along with near field techniques mitigated reflection effects. Meaningful measurements could now be accomplished in low cost non-anechoic spaces. Today FFT analyzers are smaller and cheaper than ever before and can even be made with smart phones running FFT apps.
In 1977 Berman and Fincham lamented that the “the frequency response curve, which was first used over 50 years ago, is still regarded as the primary means of assessing loudspeaker performance, although it has long been recognized that many important aspects of subjective response cannot be related to the visual appearance of the frequency response curve.”
In recognition that on-axis response alone was inadequate, a new standard, CEA2034, was released in 2013 and included specifications for 360-degree polar measurements, largely based on the techniques developed by Toole and Devantier at Harman. CEA2034 recognizes the need for a comprehensive set of acoustic data including on-axis, listening window, early reflections and sound power data. The standard recommends using a 70-point measurement along 2 orthogonal “orbits” in an anechoic chamber. Chamber design has not changed substantially since 1946, many are undersized for these measurements* and many have not been validated for polar measurements. Many organizations will need to adapt or upgrade their existing facilities to implement CEA2034. Although a big step forward, this standard favours full bandwidth frequency response data over a limited spatial resolution.
A New and Better Way
The Klippel Near Field Scanner (NFS) revolutionizes the art of loudspeaker measurement. Just as digital computers and the mathematics of FFT revolutionized acoustic measurement a few decades ago, the advanced math of spherical harmonics promises powerful characterization of the entire sound field of a speaker, quickly, in readily available, low cost spaces. Klippel’s NFS uses a holographic measurement of the sound pressure in the near field to generate coefficients to accurately determine the sound pressure at any point in the 3D sound field (outside the scanning surface).
Near field measurements have higher S/N and are less prone to reflection and air propagation effects. The scanning process can be performed in a non-anechoic environment through field separation where the outgoing wave (direct sound) is separated from the incoming waves (reflected sound). Near field scanning may be done in a “normal” room without costly acoustic treatment in a fraction of the time it would take to do similar measurements with other means. NFS generates a comprehensive data set, including a high-resolution 3D image of the sound field, with just one measurement.
SPL, directivity and other far field characteristics can be derived from the near field information. NFS is ideal for measuring the power response of large professional live performance arrays without the need for a huge chamber or the complication of precisely rotating a large and heavy DUT. The sound pressure in the near field can also be used to evaluate small studio monitors, tablets, laptops, and other personal audio devices.
The Near Field Scanner runs automatically after set-up. Initial results are available after measuring just a few points, and the measurement needs only run until the desired spatial resolution and high frequency bandwidth is reached. NFS users will enjoy more and more accurate data, more quickly using far fewer man-hours than older methods.
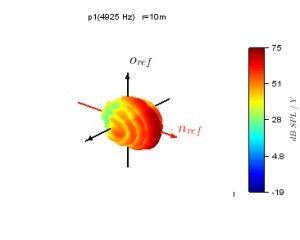
Product developers hoping to meet the 21st century’s demands to keep up with and even surpass their competitors, would be well advised to apply 21st century tools such as the Klippel NFS to their measurement toolkits.
For more information on the Near Field Scanner please contact us or visit Klippel and Warkwyn Associates at ALMA (Jan 3-4, 2015), CES (Jan 6-9, 2015, Venetian suite 31-221), or attend the Klippel Seminar in Santa Barbara CA (Jan 12-13, 2015).
* The minimum chamber dimension required to measure a full-range speaker system (1m high) accurately down to 100Hz is 5.88m (ISO3745). To measure a 1m speaker in the true far field may require a much larger chamber.
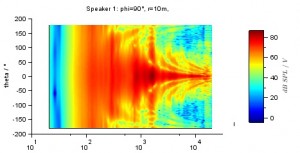
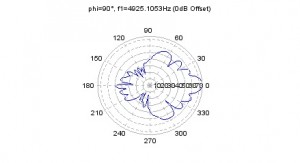
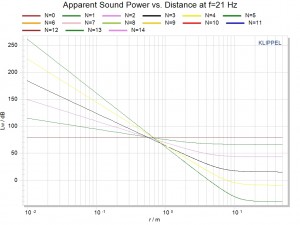
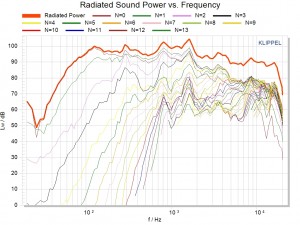
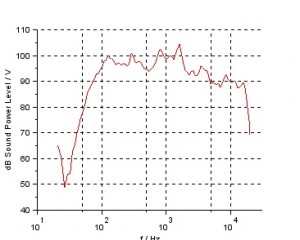
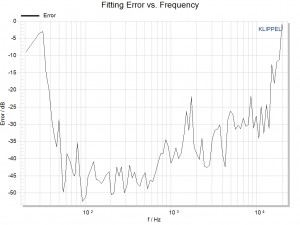
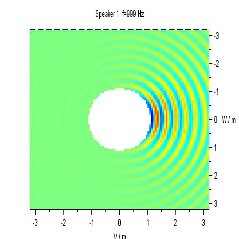